Official Guide Chemistry/Physics Section Passage 11. What stereochemical designation can be assigned to naturally occurring aspartic acid?
Amino acids are a high yield topic and we should be very comfortable with them. Come test day, we should know that naturally occurring amino acids used by all living organisms are L amino acids, and most chiral ones are S amino acids except for cysteine. Let’s look at the answer choices and see how else we can reach the correct answer.
Above you’ll see the structure of aspartic acid with the chiral carbon of interest starred. Since the hydrogen is not explicitly shown and we have an amine group pointing out of the page while the other two substituents are within the plane of the page, the hydrogen must be pointing into the page (dashed line). Whatever R/S configuration we settle upon will be the final configuration.
The nitrogen of the amine group has the greatest atomic number compared to the two yellow carbons so it will be the highest priority. We now have two carbons (yellow) so we look to what each of these carbons are immediately attached to. The carbon on the left is attached to another carbon and two hydrogens, while the carbon on the right is attached to the equivalent of 3 oxygens. Thus the carbon on the right is attached to substituents that are higher priority and the carbon on the left is lowest priority. Drawing a line from 1 -> 2 -> 3, we have a counterclockwise arc and an S configuration.
Based on what we know, as well as the configuration shown above, we can say that naturally occurring aspartic acid is S configuration.
Z refers to an alkene with each carbon’s highest priority substituents on the same side (think cis- and ze zame zide). The only double bonds we see are carbonyls, and we don’t apply Z and E designations to carbonyls. Moving on.
D and L designations are usually mentioned in the context of carbohydrates on the MCAT. They can also be used to describe amino acids. However, the aspartic acid we see above, like all other amino acids humans use, is an L amino acid.
As mentioned above, Z and E designations are not appropriate for amino acids as they lack a C=C bond. Answer choice A is then our best answer.
This question can seem difficult at first glance. Yet, there are a few different ways to reach the same, correct answer. First, the question stem asks specifically about transaminases. We know that the “-ase” ending refers to an enzyme, so the “amin” will have to tell us what functional group the enzyme uses. “Amin” looks a whole lot like “amine”, so we’ll look for the amino acid that has an amine group in its side chain.
We can also go back to the passage. The second paragraph reads “All transaminases appear to follow a common reaction mechanism illustrated in Figure 2 and utilize the same prosthetic group, pyridoxal phosphate (Compound 5). Pyridoxal phosphate acts as a carrier of the amino acid NH2 group during the reaction.” So if the transaminase uses pyridoxal phosphate, and pyridoxal phosphate carries an NH2 group, then it follows that the transaminase uses an NH2 group.
Let’s say we didn’t pick up on the enzyme name or are looking for a visual representation of the amino acid. We can look at the first step in Fig. 2 and look at what is attached to the enzyme.
We see that attached at the end of the enzyme, there is an NH2 group. While here we’re working through three different routes to reach the same answer, you would only use one of these on test day to save time. Now that we know that we’re looking for an amino acid with an amine group in its side chain, let’s look at the answer choices.
Valine is a hydrophobic amino acid with a three carbon side chain in “V” formation.
Aspartic acid is an acidic amino acid, meaning its side chain should be one that readily loses a proton. Aspartate has a carboxylic attached to another carbon as its R group.
Phenylalanine is easily recognizable due to its benzene ring attached to a carbon within the side chain. No amine group here either.
Lysine is a basic amino acid that can readily accept a proton. In particular, lysine has a single amine group following a four carbon chain as its R group. This is the answer we’ve been eagerly awaiting!
This question is testing our knowledge of Le Chatelier’s principle. Before moving on to the answer choices, we need to recognize that compounds 1 and 2 are the reactants, and compounds 3 and 4 are the products. The question is then asking what would cause an increase in the production of products.
Compound 3 is a product. According to Le Chatelier’s principle, adding a product would cause a leftward shift to restore equilibrium. This is the opposite of what we want.
Temperature can be tricky. To know the effect that temperature would have on a reaction, we would need to know whether the reaction is endothermic or exothermic. If it were an endothermic reaction, then this would be an appropriate answer choice because more product would be formed. However, because we’re not given this information, we need to keep going.
Adding more catalyst will not change the amount of product made, but instead will increase the rate or speed at which it’s made. They almost got us on this one.
Compound 2 is a reactant. According to Le Chatelier’s principle, adding more reactants causes the reaction to proceed forward and increase the amount of product made to restore equilibrium. This is the best answer choice.
The question stem is sending us to Fig. 1. The only component of the image that might give us a hint as to the thermodynamic properties of the reaction would be the equal magnitude double-headed arrows, indicating equilibrium.
While the question stem sends us to Fig. 1, reading each of the figure descriptions before moving on to the questions also indicates that Fig. 2 is just the intermediate steps within the same reaction. The Keq~1.0 mentioned with Fig. 2 must then also apply to Fig. 1. A Keq of 1, like the bidirectional arrows of equal magnitude seen in Fig. 1, points us to thermodynamic equilibrium.
As noted above, the reaction is in thermodynamic equilibrium. ATP is neither used nor produced.
ΔG=-RTlnKeq and ln(1)=0 so given that Keq~1 for this reaction, ΔG=0. At equilibrium, ΔG= 0 and no ATP is used or produced. This is exactly what we were looking for.
Because the reaction is at equilibrium, ΔG must be 0 and ATP is not involved.
This statement is factually incorrect. If ΔG>0, energy/ATP would be required for the reaction to proceed forward as it would be nonspontaneous. We’ll stick with answer choice B.
Here’s another Le Chatelier’s Principle question, this time in disguise. The question is asking us what would react with Compound 11, now a reactant, to regenerate Compound 5, now a product. In order to form more products, we can add more reactants. Compound 12 is shown so we can compare its structure to each of the answer choices.

We know this reaction is thermodynamically at equilibrium so ATP is not needed, and Compound 12 does not resemble ATP.
The figure description for Fig. 2 tells us that it is the intermediate steps for the reaction in Fig. 1. When we take a look at Fig. 1, we see that we are given the structure for oxaloacetate. The region that is similar to compound 12 is highlighted.
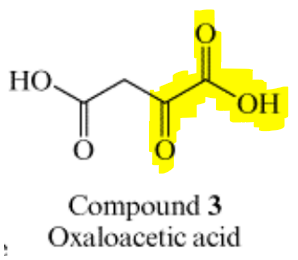
NADH is involved in redox reactions and does not structurally resemble Compound 12. Answer choice B is a better answer.
FAD+ is involved in redox reactions and does not structurally resemble Compound 12. We’re sticking with answer choice B.
The passage says “The deflection of the cantilever was measured using visible laser light…” The correct answer choice will have to fall within the visible light spectrum, approximately 400nm-700nm.
This wavelength falls below the visible light spectrum.
633nm is well within the visible light spectrum.
1.26 μm = 1.26*10-6 m=1260*10-9 m=1260nm, this is outside of the 400-700nm range.
3.17 μm = 3.17*10-6 m=3170*10-9 m=3170nm, this is outside of the 400-700nm range. Answer choice B was the only choice that fell within the visible light spectrum and as such is the correct answer.
The question stem does not explicitly send us to a given figure, however from our preliminary figure review before moving on to the questions, we know that Fig. 2 related force and extension distance. We also know that Work=F*d. To calculate the mechanical work done over the range in the question stem, we can simply take the area under the curve since the area under the curve is the distance (x-axis) * force (y-axis). Of note, we cannot simply take the peak force and extension distance and multiply them directly because the force is variable. The variability of the force means that we need to answer this question graphically. We could also use the spring equation for work, W= (1/2)*kx2. We’re not given k but since k has units of N/m, we can equate it to W=(1/2)(F/x)x2=(1/2)Fx , the same as the area of a triangle/the area under the curve.
This answer choice is orders of magnitude off. 10–22 << 10–19
While not as far from the answer we calculated above, it’s not quite right either.
This is exactly what we calculated.
This answer choice is larger than what we calculated so we’ll stick with answer choice C and move on.
Once again, the question stem does not send us to a specific figure, however Fig. 3 can help us answer this question. This figure has velocity on the x-axis and force on the y-axis. Well, Power=F*v so we can use the graph to determine the force when the speed is 1,000 nm/s and then multiply that speed by the force to get power.
The power here is off. Surely we can find an answer choice that is closer to what we calculated.
This is exactly what we calculated, love to see it!
We might arrive at this answer choice if we forgot to convert pN to N and just plugged it into the power equation, and possibly made an arithmetic error as well. Remember to convert force units to N.
We might arrive at this answer if we forgot to convert both sets of units in addition to making an arithmetic error. When plugging values into physics equations, SI units are generally our friend. We’ll stick with answer choice B.
Let’s take a moment and acknowledge that this can be a really difficult question. Now that we’ve done that, we’ll brush ourselves off and get right back to it! The question is asking for units for the rate constant. But first, what’s the rate? Well, the concentration of unfolded protein is increasing by some amount per unit of time, so out rate units are M/s. Now we have to ask ourselves, is this a zero, first, or even second order mechanism? The protein isn’t spontaneously unfolding so it’s not zero order. The figures showing us the unfolding have also been linear, so it should be a unimolecular mechanism, that is, first order.
M/s = (units for k) * (M) => units for k = (M/s)*(1/M) = (1/s)
This answer choice would represent a second order rate.
A rate constant has to have time in the denominator so this is incorrect regardless of the rate order.
This answer choice would be correct if the unfolding were zero order.
This answer choice represents a first order rate and is exactly what we’ve been looking for.
The key to this question is the question stem itself. While the mention of Ca2+ transport might make us think that we need to immediately go to Table 1, we should actually be more interested in Fig. 1. We know that Ca2+ transport changes with changing pH. The question is asking us what would account for that change in pH. When we read each of the answer choices, we realize that they all have something to do with the structure of the macrocycle so Fig. 1 is what we’ll reference first.
The figure description for Fig. 1 indicates that the X group is “=CO2H” which is a carboxylic acid functional group. We need to be comfortable recognizing basic functional groups for the MCAT. If this is something you know you’re struggling with, jot down a note to review functional groups later. A carboxylic acid will deprotonate under the majority of the pH range we’re given in the passage (pH 2-9) and deprotonation changes the pH, so we have a strong contender.
I don’t know about you, but I haven’t seen the functional group “Y = C(=O)N(n-Pr)CH2CH2OCH2CH2N(n-Pr)CO2CH2C6H5” before… I see a carbonyl, a nitrogen and some carbon chains, but nothing I immediately recognize as acidic or basic. While we may not recognize the functional group as a whole, we can say that it’s unlikely to change protonation states and if it doesn’t become protonated or deprotonated, then it shouldn’t really change the pH. This answer choice isn’t too convincing, we like answer choice A better.
Similar to the Y groups, we have no reason to believe that the oxygens within the macrocycle ring will change protonation state at the pHs we’re given. In fact, those ethers (R-O-R’) would have to be quite basic to be protonated between pH 2-9, and we know that ethers are not that basic. Let’s see what the next answer choice is.
While the passage mentions that the macrocycle binds to ions based on their radii, this does not impact the pH. Answer choice A remains the best answer.
Let’s remind ourselves of how to determine absolute configurations. We look at a chiral center and number the attached groups based on priority, specifically atomic number/molecular weight. The larger the value, the higher the priority. If two atoms immediately attached to the chiral carbon have the same priority (as in the case of the yellow carbons below), we look to see what else those atoms are attached to. The atom (in this case yellow carbon) with the greatest sum of priority groups attached to it will be assigned the higher priority. If present, hydrogen will be the lowest priority group and should point into the page or be within the plane of the page. If the lowest priority group is pointing out of the page, the configuration we determined must be reversed. Once we’ve assigned priorities to each of the unique attachments, we follow them from 1 to 4. If the arc is clockwise, it’s R configuration. If the arc is counterclockwise, it’s S configuration. Time to show what you’ve learned!
Figure 1 Structure of a synthetic macrocyclic ion transport agent featuring the 18-crown-6 backbone. (Note: X = CO2H, Y = C(=O)N(n-Pr)CH2CH2OCH2CH2N(n-Pr)CO2CH2C6H5)
Quick recap:
Left side: X group has been replaced with the functional group and we drew in the hydrogens so we could see which were pointing out of the page and which were pointing into the page. We number the substituents and get an S configuration, however we flip it because the lowest priority group is pointing out of the page.
Right side: Y group has been replaced with the functional group and we drew in the hydrogens so we could see which were pointing out of the page and which were pointing into the page. We number the substituents and get R configuration; no need to flip it because the lowest priority group is pointing into the page.
This is exactly what we obtained. The chiral center attached to X is R configuration and the chiral center attached to Y is R configuration.
The chiral center attached to Y is R configuration.
The chiral center attached to Y is R configuration. If we were tempted by this answer choice it was because we forgot to flip the configuration for the X group.
This answer choice has both chiral centers inverted. The correct answer choice is A.
The passage tells us that “Compound 1 was dissolved in an organic liquid layer serving as a membrane that separated the aqueous “IN” and “OUT” solutions.” If compound 1 is located in the organic layer, it should be primarily hydrophobic, which we can also say is lipophilic. We may need some additional information on structure, so we can remind ourselves that the functional groups on the macrocycle were
“X = CO2H, Y = C(=O)N(n-Pr)CH2CH2OCH2CH2N(n-Pr)CO2CH2C6H5.”
We know that the macrocycle as a whole is lipophilic, not hydrophilic.
The macrocycle as a whole is lipophilic, not hydrophilic.
For this we’ll take a look at the X and Y groups written above. Carboxylic acid, group X, is not nonpolar, but rather polar. This can’t be the correct answer choice because it says only nonpolar.
This answer choice correctly identifies the macrocycle as a whole as lipophilic, and we know the X group is polar. It’s safe to assume that the Y group is nonpolar given all of those carbons. Answer choice D must be the correct answer.
The last sentence of paragraph one says “The central portion of this molecule, known as a crown ether, selectively binds to cations based on their ionic radii.” If we want to favor the transport of Na+, we need to determine how the radii of the two ions compare. According to the periodic table, Na+ is in period 3 and K+ is in period 4. Even when they’ve both lost a proton, they K+ will still be in the period below Na+. Following the periodic table trends, K+ will be larger than Na+. In order to favor the smaller Na+, we will need to make the central portion of the macrocycle smaller.
The X group is not within the central portion of the macrocycle and exchanging the X group with a smaller functional group will not make the ether ring component smaller.
The Y group is not within the central portion of the macrocycle and exchanging the Y group with a smaller functional group will not make the ether ring component smaller.
Increasing the ring size would favor the transport of a larger cation, not the smaller Na+. This answer choice is the opposite of what we want.
Decreasing the size of the ring would favor the smaller Na+; this is the correct answer.
After first reading this question, many of us would find ourselves heading back to the passage to look at Table 1. After all, this is where the relationship between pH and rate of K+ transport is shown. Yet, the key to answering this question is in the question stem itself! In fact, all we need to know comes from a single word – saturated. If a transporter is saturated, it means every spot that it has available for transport is taken, in use. If the transporter is saturated, it doesn’t matter if we add one more, fifty more, or even a thousand more ions! It has no more space, and its ability to transport is maxed out. We want a graph that shows that no matter how much K+ we add, the rate of transport stays the same.
This answer shows that as more K+ is added, the rate of transport goes down. We’re looking for no impact from the additional ions.
This answer choice shows the rate increasing K+ is added. This could be true if the transporter were not saturated but cannot be true when the transporter is saturated.
Like B above, this answer choice shows the rate increasing K+ is added. This could be true if the transporter were not saturated but cannot be true when the transporter is saturated.
This answer choice correctly shows that the transporter is saturated, and that adding K+ does not impact the rate of transport.
The rate of TNBS reaction with outer envelope PE molecules is:
If you still aren’t convinced about the benefit of rephrasing question stems, allow me to try again. This question stem is long, and on test day I might find myself rereading it a few times while going through the answer choices. But, what the test makers are asking can be rephrased as simply as “what makes TNBS special? Why did the researchers like TNBS for this experiment?”
To answer this question, we can look at the second sentence of the passage: “Upon reaction, TNBS-labeled PE molecules are frozen on the outer envelope of membranes and do not exchange with other PE molecules on the inner envelope.” This means that the useful or “special” property TNBS possesses is that once it binds to the outer membrane, it yells FREEZE! like a bad action movie and nothing else can happen, the inner membrane is stuck inside, and the outer membrane is stuck outside. We want an answer choice that aligns with this.
This answer choice makes sense in the context of TNBS yelling freeze!. If the TNBS binds the outer membrane faster than the inner and outer membrane can trade places, then when TNBS freezes the membrane, we’ll get an accurate representation of the inner vs outer membrane. Let’s keep going just in case.
Right before this we said that TNBS should react quickly with the outer PE molecules in the membrane. If it were slower than the phosphate transport across the membrane, it wouldn’t be very good at “freezing” the membrane and the incubation period wouldn’t matter much. This answer choice is heading in the opposite direction of where we’re trying to go.
There’s no mention of TNBS binding to the membrane better when we add phosphate. Answer choice A is still a much better fit.
The researchers didn’t measure or mention anything that would make us think of kinetics and zero, first or second order reactions. Not today test writers… We’ll stick with answer choice A.he researchers didn’t measure or mention anything that would make us think of kinetics and zero, first or second order reactions. Not today test writers… We’ll stick with answer choice A.
We know what a phosphate ion looks like and trinitrobenzene sulfonic acid (TNBS) is shown on the right from Reaction 1.
Without looking back up to the structure of TNBS, we know that the very polar and charged nature of phosphate ions mean they are hydrophilic, not hydrophobic. They will interact with water rather than lipids.
While phosphate is hydrophilic, we need to evaluate the structure of TNBS before labeling it hydrophobic. TNBS has a benzene ring base, however it also has polar groups spread across that same ring, as well as a negatively charged attachment. The multiple polar and even charged groups mean TNBS is hydrophilic. The key here is to take into account the contribution and spread of functional groups when determining the properties of a molecule. Since TNBS is hydrophilic, this answer choice is incorrect.
For the same reason we got rid of answer choice A, we can get rid of answer choice C. Phosphate is hydrophilic, not hydrophobic.
As noted above, TNBS is hydrophilic and phosphate is hydrophilic. Time to choose answer choice D and see what the next question has in store.
PE is a lipid that forms a lipid bilayer as shown in Fig. 1. This means there should be a polar and a nonpolar region, and that the nonpolar region should include long nonpolar chains, or acyl chains. Hopefully this description is starting to remind you of the phospholipid bilayer in eukaryotic cell membranes.
Representative bilayer excerpt from Fig. 1. Notice that the PE molecules are stacked in a very familiar fashion-like the phospholipid bilayers within our own cell membranes.
In reaction 1, we can see that the polar head of the PE molecules is already accounted for (this is where the TNBS binds). This means that R1 and R2 should be the long hydrocarbons to make an acyl chain.
Be on the lookout for long carbon chains.
While carbon-based, this methyl group is much too short to serve as our long hydrocarbon chain.
This is neither carbon-based nor long.
This relatively long chain is a hydrocarbon and when attached to the carbonyl in the diagram, will make an acyl chain! Looking good so far.
While also long, this answer choice has oxygens associated with the carbons. This isn’t a hydrocarbon so we’ll go with C.
Before going to the answer choices we should know, what would be “naturally occurring” for phosphorus? A quick glance at the periodic table tells us that phosphorus has an atomic mass of 31, not 32. The correct answer will be one that increases the atomic mass of phosphorus.
This is the opposite of what we want; removing a neutron would decrease the atomic mass.
Adding a proton would change the identity of the element and would give us sulfur.
Adding electrons would change the charge, but not the atomic mass. Compared to protons and neutrons, the mass of an electron is negligible.
Adding a neutron would indeed increase the atomic mass of the phosphorus. In fact, this should ring a bell-it’s beta minus decay and the resulting emission of the beta particle will allow for the radioactivity we’re looking for!

Woah. Someone please tell this question writer they can have their coffee before coming to work. Jokes aside, this question is difficult. The two “NOT”s, no matter how large they make them, make this question difficult. Let’s start by rephrasing the question stem. We have two options: 1) What would show that the 32P is NOT entering PE molecules via direct exchange? or 2) What would support that the 32P is entering PE molecules via synthesis? On to the answer choices.
If we added TNBS before the phosphate, the membrane would freeze before the 32P could be incorporated via either mechanism. This doesn’t help us answer the question.
Acellular PE, that is PE not associated with a cell, wouldn’t be able to synthesize new PE. If we saw 32P incorporation with acellular PE, this would support direct exchange and if not, we’d have evidence against direct exchange and possible support for synthesis. We might have our answer folks.
The cell type is not what we’re questioning here, but rather the role of synthesis in the incorporation of the 32P. Changing the cell line gets us no closer to our answer. Next.
Decreasing the concentration of phosphate would mean less 32P is available for either mechanism of incorporation, and would not help us determine which mechanism is more likely. For this monster question, we’ll stick with answer choice B.
In the second sentence of the first paragraph, the passage identifies the hardness ions as “Ca2+, Mg2+, and Fe2+.” Beyond that point, it lumps them all together as the generic M2+.
The alkaline earth metals are in group 2 of the periodic table. Iron (Fe) is not in group 2 and not an alkaline earth metal.
The cations of weak bases have acidic properties. However, this means that the alkali and alkaline earth metals (two of the three hardness ions above) which make for strong bases (think Mg(OH)2), do not produce strongly acidic cations. This cannot be the correct answer.
Reduction is the gain of electrons. The hardness ions above have lost electrons compared to their elemental state, not gained them.
We just established that the hardness ions above have lost electrons. We can be even more specific and say that they’ve each lost two electrons because their charge is 2+. Answer choice D it is!
What happens to the pH of a soapy solution as a result of the introduction of hardness ions?
This answer choice is factually incorrect. An increase in proton concentration would cause a decrease in pH. Recall that pH=-log[H+].
An acid-base reaction does occur. This answer choice might be tempting because we don’t see frequent mentions of acids and bases the way we normally see in acid-base chemistry passages. However, in the second paragraph, the author mentions that the hardness ions “reduce the effectiveness of common soaps, which contain the sodium salts of organic acids,” indicating that the hardness ions are binding to and removing the conjugate bases that make up the soap out of solution which would indeed alter the pH.
As mentioned in the explanation for answer choice B, the hardness ions are removing weak bases out of solution. This means that the pH should decrease and [OH-], representing the base concentration in solution, should decrease. Recall that water autoionizes into hydronium and hydroxide anions. If we have an aqueous solution, we have both present, even when they’re not explicitly included in the chemical equation.
If the identity of the cation mattered, the author would not have used the generic form M2 for each of the reactions. The effect on pH will be the same for each of the cations. Answer choice C remains the correct answer.
This has the potential to be a pseudodiscrete-we’re expected to know the basic organic chemistry behind soaps and their functional groups. That said, the passage includes a very helpful line: “effectiveness of common soaps, which contain the sodium salts of organic acids with long carbon chains.” The question asks which is not a precursor to soap, so we’re looking for a structure that lacks a long carbon chain.
Fatty acids are known for their long carbon chains attached to a carboxylic acid; they can be used to make soap.
Cholesterol is composed of four fused carbon rings. We don’t expect these fused rings to make soap so B is looking good but we’ll continue just in case.
Triacylglycerols, like fatty acids, contain long carbon chains and can be used to make soap.
Phospholipids, like triacylglycerols and fatty acids, contain long carbon chains and can be used to make soap. Of all of the answer choices, B, cholesterol, is the only one that cannot be used to make soap.
This is a long question stem. Let’s break it down. A pipe has boiler scale. The passage tells us that boiler scale causes the pipe radius to constantly decrease with time. The question is then asking about the energy needed to maintain constant flow through a pipe with a progressively decreasing radius.
Thinking about this conceptually first, we can imagine that the smaller the hole, the harder it is for water to flow through that hole. Now think about a water hose in your backyard. The flow rate through the water hose is set by how far you open the dial or pump and must remain constant. When you place your finger over part of the hose outlet, the water that comes out does so more forcefully because in order to maintain constant flow, a decrease in radius will correspond to an increase in pressure to the fourth power. Using pressure as a surrogate for energy for this particular question stem, we would expect more energy to be required in order to get the same amount of water flowing out of the hose in the same period of time through a smaller radius.

Now, let’s take a look at Poiseuille’s law. The relationship between the radius and the pressure is not linear. For everything else to stay constant, a single change in radius requires an exponential change in pressure, so the energy required to maintain flow should be exponential!

This answer choice incorrectly shows the energy requirement decreasing with time/decreasing radius.
This answer choice incorrectly shows the energy requirement increasing linearly with time/decreasing radius. As evidenced by our sample calculation, the pressure and energy requirement should be increasing exponentially.
This answer choice shows an initial exponential increase in energy requirement, but then plateaus. The plateau would mean that once the hole is small enough, the amount of energy required to maintain flow stops increasing. But, based on Poiseulle’s law, the more we decrease the radius, the more pressure/energy is required to maintain flow. There’s nothing to show that the energy needs change once the radius reaches a certain size. Let’s look at D.
Answer choice D correctly shows the energy requirement increasing exponentially with time/decreasing radius. This is what we’ve been looking for and there are no unexpected changes shown with different radii. This is therefore the best answer choice.
Dissolve the solid in a known volume of:
If we want to dissolve the precipitate, we must first understand what the precipitate is composed of, in this case a hardness ion and a weak conjugate base, making the soapy precipitate the salt of a weak base.
The common ion effect states that when a new solute is added to a solution that shares a common ion, the solubility of the new solute decreases. This is a specific instance of Le Chatelier’s Principle and explains why we don’t dissolve acids in acids or dissolve bases in bases. Instead, we dissolve acids in bases and bases in acids. This allows some of the products to neutralize each other and shift the reaction forward, actually increasing solubility!
The soapy precipitate is a base, so we would dissolve it in an acid. On to the answer choices.
NaHCO3 is a base, and we would not successfully dissolve the soapy precipitate.
NaOH is a strong base, and we would not successfully dissolve the soapy precipitate.
NaCl is neither an acid nor a base. Additionally, titrating a base with another base will make the pH increase without allowing us to obtain an equivalence point. This wouldn’t work either
Ah, this is much better. By dissolving the precipitate in an acid, we increase solubility and will have an overall acidic solution of free fatty acids to titrate. Since the solution we’ll be titrating is acidic, we will titrate and neutralize it with a base, in this case NaOH. By approaching this question systematically, we can arrive at D as our final answer.
To find the ΔG° for glycolysis as a whole, we’ll need to take the sum of the two separate processes. Let’s write this out:ΔG° glycolysis = ΔG° reaction 1 + 2* ΔG° reaction 2
Why are we adding double the ΔG° for reaction 2? The reason is that the overall reaction produces two ATP molecules and the ΔG° we’re given for reaction 2 is only for one ATP molecule. Back to the math:
ΔG° glycolysis = ΔG° reaction 1 + 2* ΔG° reaction 2
ΔG° glycolysis = (-146 kJ/mol) + 2*(30.5 kJ/mol) = -146 kJ/mol + 61 kJ/mol
= -85 kJ/mol
The magnitude of this answer choice is too large. If we got this number, we likely changed the sign of the ΔG° for reaction 2.
The magnitude of this answer choice is too large. If we got this number, we likely changed the sign of the ΔG° for reaction 2 and did not account for the second ATP produced.
This is the answer we calculated above and is the correct answer.
This answer choice is incorrect because it adds the ΔG° for a third ATP molecule, but only two are produced in the net glycolysis reaction.
A helium nucleus, or alpha particle, is emitted during alpha decay.
Alpha absorption is the opposite of alpha decay: in alpha absorption, a helium nucleus is absorbed by the atom, not emitted.
If gamma decay emits gamma ray photons, gamma absorption must involve an atom absorbing gamma ray photons.
There are two main types of beta decay, beta plus and beta minus, which emit positrons and electrons respectively. Both are considered beta particles. The MCAT sometimes distinguishes between beta plus and beta minus decay in the answer choices, and sometimes it lumps the two together as it does in this answer choice. Be familiar with the differences but also recognize that an answer choice can refer to either/or. Beta decay is the correct answer for this question and results in the emission of positrons.
Before getting overwhelmed by the shape of that curve, let’s break this figure down into its basic components. You know the drill:
1) read the figure description, title, etc.
2) read the axes and note any applicable units; for C/P questions in particular (but not exclusively!) determine if you know any equations that relate the two axes
3) take a look at the general trends and results
4) determine significance as appropriate1) There’s no title, but the question stem tells us that it is a pressure-volume loop.
2) The x-axis is “Intrathoracic pressure” with units of “cm H2O” and the y-axis is “Lung volume” with units of “L(iters).” Let’s pause, do we know any equations that relate pressures and volumes? Absolutely! Two of the most important pressure-volume equations on test day are the ideal gas law (PV=nRT) and the work equation in thermodynamics (W=PV). We haven’t looked at the answer choices yet so we’re not sure which would be helpful here, but both equations multiply pressure and volume, which correspond to the area under the curve because at its core, the area under the curve is the x-axis * y-axis which we adjust as needed for shape.
3) The curve is oblong and because we’re asked about the area in the curve and not the processes outlining the changes (expiration and inspiration) we’ll stop there.
4) Significance is not applicable here.
The work equation we identified earlier corresponds to the very first answer choice, looking good! You might be thinking “Wait! But the pressure on the curve is in cm H2O, not in Pascals!” You’re absolutely correct. That said, cm H2O can be converted to Pascals via a simple conversion factor, and it represents the same measure, pressure. You’ll learn a bit more about measuring pressures in cm H2O in medical school, so don’t worry about it too much for now (I’m looking at you respiratory system…).
While tempting because we know that inspiration and expiration move oxygen, there are two main reasons this answer choice is incorrect. The first is that inspiration and expiration do not only move oxygen; we have to move and remove CO2 as well! The other, more important reason is that the units of oxygen quantity are inconsistent with the unit of work. The former would have a volume unit (L, mL, m3) while the latter is derived from volume and pressure multiplied (J).
This is just the y-axis, not the area under the curve.
This is just the x-axis, not the area under the curve. Answer choice A is the only answer that correctly identifies the relationship between the pressure and volume shown in the figure.
The first sentence in the question stem doesn’t tell us a whole lot so we’ll focus on the second sentence. We want to know what measure affects frequency. Time to use the wave equation:
Velocity = wavelength * frequency
The vocal cords aren’t changing length in your body, they are anchored which means that wavelength is fixed. That really only leaves us with velocity affecting frequency. This is where things get a bit sticky. Sound, as it moves through air, is a longitudinal wave. However, when a wave propagates through the vocal cords to produce the sound waves, the wave on the vocal cords is a transverse wave. What might affect the speed, and therefore the frequency, as a transverse wave travels along the fixed-length vocal cords?
Speed is independent of volume, so this would be incorrect.
The speed of a wave traveling down a string is determined by two key factors, tension and density: v =√T/ρ . This means that a change in density would affect the speed at which the wave travels and would influence the frequency of the sound produced. The reason that C is a better answer choice as we’ll see next, is that tension is a property that can easily be changed as the vocal cords produce sound, but density is not easily changed. Our vocal cords cannot spontaneously become more or less dense in the same breath, so while tempting, density is not the best answer choice for this question.
As noted above, both density and tension affect the speed of a wave propagating down a string. That said, tension is easier to change than density. Imagine you and a friend are jumping rope, each holding a different end of the rope. Now, instead of moving the rope around in circles, you lift your end of the rope up and down, sending waves to your friend. If you stand really close to your friend and hold the rope with very little tension, those waves don’t travel very fast… However, if you stand further away and increase the tension in the jump rope, the waves you send down the rope are pretty quick, and the change was easy to make! Tension provides a solid explanation for a change in velocity, so it could easily affect frequency. This will be the correct answer choice.
The number of vocal cords in our larynx does not change when we talk, so this is incorrect. We’ll stick with answer choice C and move on to the next question.
(Note: Use 3 m/s for the speed of sound through the vocal cord.)
The vocal cords are fixed at both ends, so the standing wave equation we use needs to correspond to an open pipe, recalling that an open pipe has two open/free ends or two closed/fixed ends. The question stem asks for the fundamental frequency, so n=1.
Frequency = (n*velocity)/(2*length)
Frequency = (1* 3m/s) / (2* 15*10-3 m) = (3 m/s) / (30*10-3 m) = 1/10-2 s-1 = 100 HzUnderstanding what affects wave velocity can be difficult to understand at times. The corresponding content page is a great start: https://jackwestin.com/resources/mcat-content/periodic-motion/transverse-and-longitudinal-waves-wavelength-and-propagation-speed
This answer choice is an order of magnitude too big, make sure you used the unit mm and not cm when plugging in the vocal cord length.
This is the correct answer; we worked out the math above.
Double check your orders of magnitude. Did you by chance make the speed of sound 30 m/s or the vocal cord length 1.5 mm?
This answer choice is 100x larger than the correct answer, double check the units and associated orders of magnitude here. This answer choice is most likely a combination of plugging in a larger velocity and a smaller length.
This question is asking about the behavior of enzymatic rate as a function of substrate concentration. This should ring a bell in the form of a Michaelis-Menten curve! As more substrate is added, the rate of the reaction increases. However, given a limited number of enzymes, there will eventually be a point where the enzymes are saturated and all of their catalytic sites are full, so adding more substrate will not cause an increase in the rate. The correct answer should have an initial increase in rate as substrate is added, and then level off and stop increasing at some point once saturation is achieved.
This is the curve we were looking for: there is an initial increase in the enzymatic rate as substrate is added and then we reach a plateau where adding more substrate does not impact rate because the enzyme is saturated.
This curve does not account for eventual saturation. It also assumes that the rate will increase linearly, but it will not. Think of it this way: when there are very few substrate molecules but a lot of enzymes, those enzymes will quickly bind the substrate and catalyze the reaction. However, as more substrates bind the enzymes, fewer enzymes and catalytic sites are available, so it takes longer for a substrate molecule to bind the enzyme. The increase in rate is not 1:1.
This curve does not address the possibility of saturation and actually shows the rate increasing as more of the enzymes are bound to substrate and more substrate is added, rather than decreasing the way it should as less catalytic sites are available for binding.
As more substrate is added, the rate of reaction catalysis should increase, not decrease. Answer choice A is the best curve.